What Is Carbon Fiber?
Table of Contents
- What is Carbon Fiber?
- What Does Carbon Fiber Look Like?
- Creating Carbon Fiber Composites
- Properties of Carbon Fiber
- Comparing Carbon Fiber to Aluminum and Steel
- High Modulus Carbon Fiber
- Potential Performance of Carbon Fiber Composites
- What is a Carbon Fiber Composite Sandwich Structure?
- Pros and Cons of Carbon Fiber Composites
- Carbon Fiber vs. Metals
- Carbon Fiber Engineering for Custom Applications
Carbon fiber is a material consisting of thin, strong crystalline filaments of carbon, essentially carbon atoms bonded together in long chains. The fibers are extremely stiff, strong, and light, and are used in many processes to create excellent structural materials. Carbon fiber offers a variety of benefits including:
- High stiffness and stiffness-to-weight ratio
- High tensile strength and strength-to-weight ratio
- High-temperature tolerance with special resins
- Low thermal expansion
- High chemical resistance
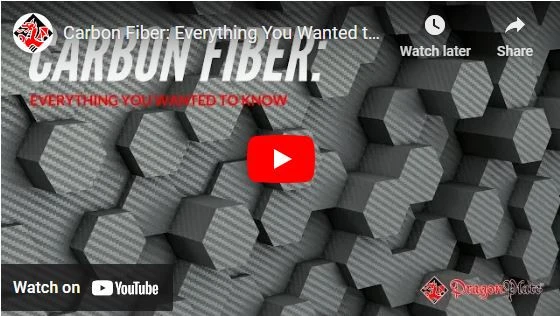
What Does Carbon Fiber Look Like?
Carbon fiber is made in black strands or yarns called "Tows" and comes in a variety of formats, including spools of tow, unidirectional formats, weaves, braids, and others, which are used to create carbon fiber composite parts.
Within each of these formats are sub-categories of further refinement. For example, different carbon fiber weaves can result in different properties in the composite part.
What Material is Carbon Fiber?
Carbon fiber is a material made from thin filaments of carbon atoms bonded together in a crystalline structure, which results in an exceptional strength-to-weight ratio. The manufacture of carbon fiber material involves several complex steps that transform carbon-rich precursors into spools of fibers.
Most carbon fibers are produced using polyacrylonitrile (PAN), pitch, or rayon as the precursor. The selected precursor material is first spun to create long fibers. The raw fibers are then heated in an oxygen-free environment in a process called stabilization to prevent them from burning or shrinking. After stabilization, the fibers are carbonized at a very high temperature of around 1000 - 3000 degrees Celsius in an inert atmosphere such as nitrogen. This drives out almost all non-carbon elements from the fibers leaving behind almost pure carbon atoms in a crystalline structure. Finally, the fibers receive a surface treatment to enhance their adhesion to matrix materials and then wound onto spools.
Creating Carbon Fiber Composites
To create a composite part, the carbon fibers, which are stiff in tension and compression, need to be supported in a stable matrix to maintain the part shape. Epoxy resin is an excellent plastic with good physical properties and is often used for this matrix, with carbon fibers providing strength.
Since both epoxy and carbon fiber are low-density, one can create a part that is lightweight, but very strong. When fabricating a composite part, a multitude of different processes can be utilized, including wet-layup, vacuum bagging, resin transfer, matched tooling, insert molding, pultrusion, and many other methods. In addition, the selection of the resin allows for tailoring specific properties such as elevated temperature or chemical resistance.
Carbon fibers reinforcing a stable matrix of epoxy
Properties of Carbon Fiber
Carbon fiber is extremely stiff, strong, and light. It is typical in engineering to compare the properties of materials in terms of their strength-to-weight ratio and stiffness-to-weight ratio, particularly in structural design, where added weight may translate into increased lifecycle costs or unsatisfactory performance.
Modulus of Elasticity and Tensile Strength of Carbon Fiber
The stiffness of a material is measured by its modulus of elasticity. This is very similar to Spring Rate, a metric used to describe the stiffness of springs. It is calculated by dividing the change in stress by the change in strain. The modulus of carbon fiber is typically 33 msi (228 Gpa) and its ultimate tensile strength is typically 500 ksi (3.5 Gpa).
Mechanical Properties of Carbon Fiber Composite Parts
The stiffness and strength along any specific axis in a carbon fiber composite part depend not only on fiber and resin mechanical properties, but also on fiber placement and orientation, and fiber/resin ratio (typically approx. 50/50 ratio). A typical value for the stiffness of a carbon fiber composite plate would be 10 msi, and its strength would be 90 ksi.
Stiffness-To-Weight Ratio
Plain-weave carbon fiber reinforced laminate has an elastic modulus of approximately 8 msi and a volumetric density of about 0.05 lbs./in3. The stiffness-to-weight for this material is 160 x 106. By comparison, the density of aluminum is 0.10 lbs./in3, which yields a stiffness to weight of 100 x 106. The density of 4130 steel is 0.30 lbs./in3, which yields a stiffness to weight of 100 x 106.
See table below
Material | Elastic Modulus | Volumetric Density | Stiffness-to-Weight |
Plain-Weave Carbon Fiber Composite | 8 msi | 0.05 lbs./in3 | 160 x 1066 |
6061-T6 Aluminum | 10 msi | 0.10 lbs./in3 | 100 x 106 |
Steel | 30 msi | 0.30 lbs./in3 | 100 x 106 |
Hence even a basic plain-weave carbon fiber panel has a stiffness-to-weight ratio of 60% greater than aluminum or steel.
Comparing Carbon Fiber to Aluminum and Steel
Compare the above with 2024-T3 Aluminum, which has a modulus of 10 msi and an ultimate tensile strength of 65 ksi, and 4130 Steel, which has a modulus of 30 msi and ultimate tensile strength of 125 ksi.
Yield Strength in Carbon Fiber vs. Steel
Steel will permanently deform at a stress level below its ultimate tensile strength. The stress level at which this occurs is called yield strength. Carbon fiber, on the other hand, will not permanently deform below its ultimate tensile strength, so it effectively has no yield strength.
High Modulus Carbon Fiber
Higher-stiffness carbon fibers are available through specialized heat treatment processes. The utilization of prepreg, and high-modulus or ultra-high-modulus carbon fiber prepregs, yields substantially higher stiffness-to-weight ratios. For very demanding applications where maximum stiffness is required, 110 msi ultra-high modulus carbon fiber can be used. This specialized pitch-based carbon fiber has a bending stiffness over 3 times that of a standard modulus prepreg panel (about 25 msi). When one considers the possibility of customized carbon fiber panel stiffness through strategic laminate placement, a panel (or other cross-section, such as a carbon fiber tube) can be fabricated with bending stiffness on the order of 50 msi.
Potential Performance of Carbon Fiber Composites
All zero-degree oriented unidirectional ultra-high modulus coupon samples have tensile stiffness of more than 70 msi, or over twice the stiffness of steel, yet still only half the weight of aluminum. The stiffness-to-weight ratio of this material is then over 10 times that of either steel or aluminum. When one includes the potentially massive increases in strength-to-weight and stiffness-to-weight ratios possible when these materials are paired with lightweight honeycomb and foam cores, the impact of advanced carbon fiber composites becomes obvious.
What is a Carbon Fiber Composite Sandwich Structure?
A composite sandwich combines the superior strength and stiffness properties of carbon fiber with a lower-density core material. In Dragonplate sandwich sheets, a thin carbon-fiber skin is laminated over a foam, honeycomb, balsa, or birch plywood core. By combining these materials, one can create a final product with a much higher stiffness-to-weight ratio. For applications where weight is critical, carbon-fiber sandwich sheets may be the right fit.
Composite Sandwich Stresses vs Stresses in an I-Beam
A composite sandwich structure is similar to a homogeneous I-Beam construction in bending.
Figure 1: Diagram showing carbon-fiber composite sandwich and equivalent I-Beam
Referring to the picture of the sandwich structure, at the center of the beam (assuming symmetry) lies the neutral axis, which is where the internal axial stress equals zero.
Moving from bottom to top in the diagram, the internal stresses switch from compressive to tensile. Bending stiffness is proportional to the cross-sectional moment of inertia, as well as the material modulus of elasticity.
Thus, for maximum bending stiffness, one would place an extremely stiff material as far from the neutral axis as possible. By placing carbon fiber furthest from the neutral axis and filling the remaining volume with a lower-density material, the result is a composite sandwich material with a very high stiffness-to-weight ratio.
Figure 2: Comparison of internal stress distribution for solid laminate and sandwich construction in bending.
Analysis of Birch Plywood Core Laminate vs. a Solid Caron Fiber Layup
FEA analyses comparing stress levels in a sandwich laminate vs solid carbon fiber are shown below. These calculations show the deflections of a cantilever beam with a load placed at the end. In the figure, 3/16" birch plywood core laminate is shown next to a solid carbon fiber layup of equal weight. Due to the reduced thickness of the solid carbon beam, it deflects significantly more than the equivalent beam made using a core material. As the thickness increases, this disparity becomes even greater due to the large weight savings from the core. Similarly, one can replace a solid carbon structure with a lighter one of equivalent strength and stiffness made from any of the core options previously mentioned.
Figure 3: Finite element analysis comparison between Dragonplate sandwich laminate and solid carbon fiber
Strengths and Weaknesses of the Various Carbon Fiber Sandwich Cores
When utilizing the various cores, each one has strengths and weaknesses. Typically, the driving factors are compressive and shear strength of the core. For example, if high compressive strength (and hence high crush resistance) is required, then the core will most likely need to be of higher density (high-density foam or birch plywood are good options here). If, however, one needs the absolute lowest weight composite possible, and the stresses are relatively small (i.e., low load, high stiffness application), then an extremely lightweight foam or honeycomb core may be the best selection.
Some cores offer better moisture resistance (closed-cell foam), some have better machinability (plywood), and others high compressive strength-to-weight ratio (balsa). It is up to the engineer to understand the tradeoffs during the design process to maximize the potential of cored composites.
That said, for weight-critical applications, there is often no other option that even comes close to the potential strength and stiffness-to-weight ratios of carbon fiber sandwich core laminates.
COMPARISON CRITERIA | |||||
PRODUCTS | Stiffness to Weight | Toughness | Crushability | Moisture Resistance | Sound Absorbency |
Solid Carbon Fiber | GOOD | BETTER | BEST | BEST | POOR |
High Modulus Solid Carbon Fiber | BETTER | GOOD | BETTER | BEST | POOR |
Birch Core | BETTER | BEST | BEST | GOOD | POOR |
Balsa Core | BETTER | GOOD | BETTER | POOR | GOOD |
Nomex Honeycomb Core | BEST | GOOD | BETTER | BETTER | BEST |
Depron Foam Core | BETTER | POOR | POOR | BETTER | BETTER |
Airex Foam Core | BEST | GOOD | GOOD | BETTER | BETTER |
Divinycell Foam Core | BETTER | BETTER | BETTER | BETTER | GOOD |
Last-A-Foam Core | BETTER | BETTER | BETTER | BETTER | BETTER |
Pros and Cons of Carbon Fiber Composites
Carbon fiber reinforced composites have several highly desirable traits that can be exploited in the design of advanced materials and components (for example, solid carbon sheets, carbon fiber sandwich laminates, carbon tubes, etc.). The two most common uses for carbon fiber are in applications where a high strength to weight and high stiffness to weight are desirable. These include aerospace, military structures, robotics, wind turbines, manufacturing fixtures, sports equipment, and many others.
Pros:
- Strength-to-weight ratio
- Stiffness-to-weight ratio
- Electrical conductivity
- Thermal conductivity
- Carbon fiber will not plastically yield
- Unique and beautiful surface finish
High toughness can be accomplished when combined with other materials. Certain applications also exploit carbon fiber's electrical conductivity, as well as high thermal conductivity in the case of specialized carbon fiber.
Cons:
- Once the ultimate strength is exceeded, carbon fiber will fail suddenly and catastrophically.
- Carbon fiber materials are significantly more expensive than traditional materials
- Working with carbon fiber requires a high skill level and specialized tooling to produce high-quality structural materials
- Carbon fiber will not yield. Under load, carbon fiber bends but will not remain permanently deformed. Once the ultimate strength of the material is exceeded, carbon fiber will fail suddenly and catastrophically. In the design process, it is critical that the engineer understand and account for this behavior, particularly in terms of design safety factors.
Carbon Fiber vs. Metals
When designing composite parts, one cannot simply compare the properties of carbon fiber versus steel, aluminum, or plastic, since these materials are in general homogeneous (properties are the same at all points in the part) and have isotropic properties throughout (properties are the same along all axes). By comparison, in a carbon fiber part, the strength resides along the axis of the fibers, and thus fiber properties and orientation greatly impact mechanical properties. Carbon fiber parts are in general neither homogeneous nor isotropic.
Carbon Fiber Engineering for Custom Applications
The properties of a carbon fiber part are close to that of steel and the weight is close to that of plastic. Thus, the strength-to-weight ratio (as well as stiffness-to-weight ratio) of a carbon fiber part is much higher than either steel or plastic. The specific details depend on the matter of construction of the part and the application. For instance, a foam-core sandwich has an extremely high strength-to-weight ratio in bending, but not necessarily in compression or crush. In addition, any components' loading and boundary conditions are unique to the structure within which they reside. Thus, it is impossible for us to provide the thickness of the carbon fiber plate that would replace the steel plate in your application. It is the customer's responsibility to determine the safety and suitability of any Dragonplate product for a specific purpose. This is accomplished through engineering analysis and experimental validation.